It is well known that nitrogen-containing organic compounds present in liquid or solid fossil fuel can contribute to the total NOx formed during the combustion process. This fuel nitrogen is a particularly important source of nitrogen oxide emissions for residual fuel oil and coal, which typically contain 0.3–2% nitrogen by weight. Studies have shown that most of the nitrogen in heavy fuel oils is in the form of heterocycles, and it is thought that the nitrogen components of coal are similar [285]. It is believed that pyridine, quinoline, and amine type heterocyclic ring structures are of importance.
The extent of the conversion of fuel nitrogen to NOx is dependent on the local combustion characteristics and the initial concentration of nitrogen-bound compounds. Fuel-bound compounds that contain nitrogen are released into the gas phase when the fuel droplets or particles are heated during the devolatilization stage. From the thermal decomposition of these compounds (aniline, pyridine, pyrroles, and so on) in the reaction zone, radicals such as HCN, NH3, N, CN, and NH can be formed and converted to NOx. The above free radicals (that is, secondary intermediate nitrogen compounds) are subject to a double competitive reaction path. This chemical mechanism has been subject to several detailed investigations [441]. Although the route leading to fuel NOx formation and destruction is still not completely understood, different investigators seem to agree on a simplified model:
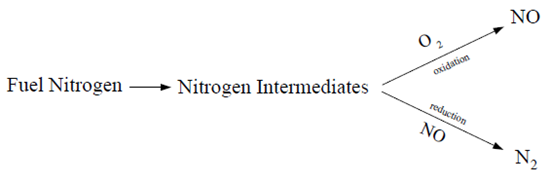
Recent investigations [257] have shown that hydrogen cyanide appears to be the principal product if fuel nitrogen is present in aromatic or cyclic form. However, when fuel nitrogen is present in the form of aliphatic amines, ammonia becomes the principal product of fuel nitrogen conversion.
In the Ansys Fluent NOx model, sources of NOx
emission for gaseous, liquid, and coal fuels are considered separately. The nitrogen-containing
intermediates are grouped as HCN, NH3, or a combination of both.
Transport equations (Equation 9–1 and Equation 9–2 or
Equation 9–3) are solved, after which the source terms ,
, and
are determined for different fuel types. Discussions to follow refer to fuel
NOx sources for
and intermediate HCN, and NH3 sources for
and
. Contributions from thermal and prompt mechanisms have been discussed in
previous sections.
The fuel NOx mechanisms for gaseous and liquid fuels are based on different physics, but the same chemical reaction pathways.
When HCN is used as the intermediate species:
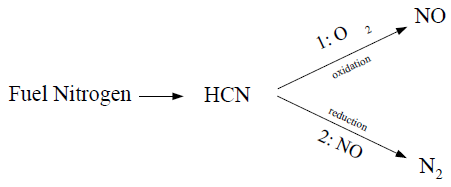
The source terms in the transport equations can be written as follows:
(9–31) |
(9–32) |
The rate of HCN production is equivalent to the rate of combustion of the fuel:
(9–33) |
where | |
| |
| |
|
The mean limiting reaction rate of fuel, , is calculated from the Magnussen combustion model, so the gaseous fuel
NOx option is available only when the generalized finite-rate model is
used.
The rate of HCN production is equivalent to the rate of fuel release into the gas phase through droplet evaporation:
(9–34) |
where | |
| |
| |
| |
|
The HCN depletion rates from reactions (1) and (2) in the above mechanism are the same for both gaseous and liquid fuels, and are given by De Soete [139] as
(9–35) |
(9–36) |
where | |
| |
| |
| |
| |
| |
| |
|
The oxygen reaction order, , is calculated from Equation 9–30.
Since mole fraction is related to mass fraction through molecular
weights of the species () and the mixture (
),
(9–37) |
The mass consumption rates of HCN that appear in Equation 9–31 are calculated as
(9–38) |
(9–39) |
where | |
| |
| |
| |
|
NOx is produced in reaction 1, but destroyed in reaction 2. The sources for Equation 9–32 are the same for a gaseous as for a liquid fuel, and are evaluated as follows:
(9–40) |
(9–41) |
When NH3 is used as the intermediate species:
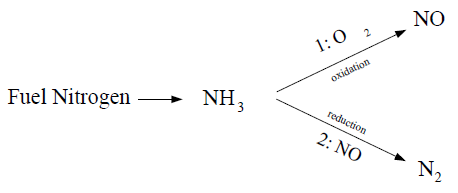
The source terms in the transport equations can be written as follows:
(9–42) |
(9–43) |
The rate of NH3 production is equivalent to the rate of combustion of the fuel:
(9–44) |
where | |
| |
| |
|
The mean limiting reaction rate of fuel, , is calculated from the Magnussen combustion model, so the gaseous fuel
NOx option is available only when the generalized finite-rate model is
used.
The rate of NH3 production is equivalent to the rate of fuel release into the gas phase through droplet evaporation:
(9–45) |
where | |
| |
| |
| |
|
The NH3 depletion rates from reactions (1) and (2) in the above mechanism are the same for both gaseous and liquid fuels, and are given by De Soete [139] as
(9–46) |
where | |
| |
| |
| |
| |
| |
| |
|
The oxygen reaction order, , is calculated from Equation 9–30.
Since mole fraction is related to mass fraction through molecular
weights of the species () and the mixture (
),
can be calculated
using Equation 9–37.
The mass consumption rates of that
appear in Equation 9–42 are calculated
as
(9–47) |
(9–48) |
where | |
| |
| |
| |
|
NOx is produced in reaction 1, but destroyed in reaction 2. The sources for Equation 9–43 are the same for a gaseous as for a liquid fuel, and are evaluated as follows:
(9–49) |
(9–50) |
For the coal it is assumed that fuel nitrogen is distributed between the volatiles and the char. Since there is no reason to assume that N is equally distributed between the volatiles and the char, the fraction of N in the volatiles and the char should be specified separately.
When HCN is used as the intermediate species, two variations of fuel NOx mechanisms for coal are included. When NH3 is used as the intermediate species, two variations of fuel NOx mechanisms for coal are included, much like in the calculation of NOx production from the coal via HCN. It is assumed that fuel nitrogen is distributed between the volatiles and the char.
The first HCN mechanism assumes that all char N converts to HCN, which is then converted partially to NO [613]. The reaction pathway is described as follows:
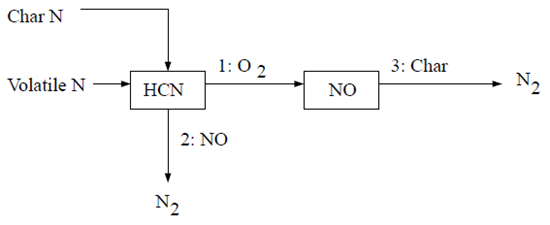
With the first scheme, all char-bound nitrogen converts to HCN. Thus,
(9–51) |
(9–52) |
where | |
| |
| |
|
The second HCN mechanism assumes that all char N converts to NO directly [387]. The reaction pathway is described as follows:
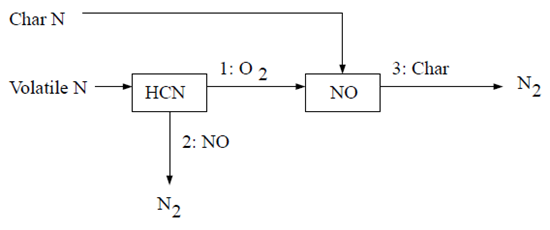
According to Lockwood [387], the char nitrogen is released to the gas phase as NO directly, mainly as a desorption product from oxidized char nitrogen atoms. If this approach is followed, then
(9–53) |
(9–54) |
The second HCN mechanism tends to produce more NOx emission than the first. In general, however, it is difficult to say which one outperforms the other.
The source terms for the transport equations are
(9–55) |
(9–56) |
The source contributions ,
,
, and
are described previously. Therefore, only the heterogeneous reaction source,
, the char NOx source,
, and the HCN production source,
, need to be considered.
The heterogeneous reaction of NO reduction on the char surface has been modeled according to the following [360]:
(9–57) |
where | |
| |
| |
| |
| |
|
The partial pressure is calculated using
Dalton’s law:
The rate of NO consumption due to reaction 3 will then be
where | |
| |
| |
|
The heterogeneous reaction involving char is mainly an adsorption process, whose rate is
directly proportional to the pore surface area. The pore surface area is also known as the
BET surface area, due to the researchers who pioneered the adsorption theory (Brunauer,
Emmett, and Teller [85]). For commercial adsorbents, the pore
(BET) surface areas range from 100,000 to 2 million square meters per kilogram, depending on
the microscopic structure. For coal, the BET area is typically
25,000 m2/k g, which is used as the default in Ansys Fluent. The
overall source of HCN () is a combination of volatile contribution (
) and char contribution (
):
The source of HCN from the volatiles is related to the rate of volatile release:
where | |
| |
| |
|
Calculation of sources related to char-bound nitrogen depends on the fuel NOx scheme selection.
The first NH3 mechanism assumes that all char N converts to NH3 which is then converted partially to NO [613]. The reaction pathway is described as follows:
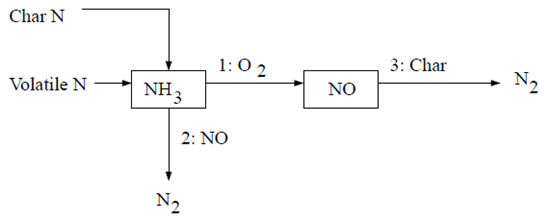
In this scheme, all char-bound nitrogen converts to NH3. Thus,
(9–58) |
(9–59) |
where | |
| |
| |
|
The second NH3 mechanism assumes that all char N converts to NO directly [387]. The reaction pathway is described as follows:
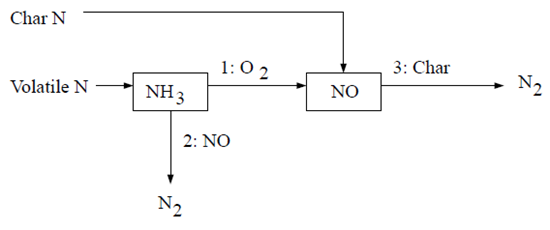
According to Lockwood [387], the char nitrogen is released to the gas phase as NO directly, mainly as a desorption product from oxidized char nitrogen atoms. If this approach is followed, then
(9–60) |
(9–61) |
The second NH3 mechanism tends to produce more NOx emission than the first. In general, however, it is difficult to say which one outperforms the other.
The source terms for the transport equations are
(9–62) |
(9–63) |
The source contributions ,
,
,
,
, and
are described previously. Therefore, only the NH3
production source,
, must be considered.
The overall production source of NH3 is a combination of volatile
contribution (), and char contribution (
):
(9–64) |
The source of NH3 from the volatiles is related to the rate of volatile release:
where | |
| |
| |
|
Calculation of sources related to char-bound nitrogen depends on the fuel NOx scheme selection.
In certain cases, especially when the fuel is a solid, both HCN and NH3 can be generated as intermediates at high enough temperatures [472]. In particular, low-ranking (lignite) coal has been shown to produce 10 times more NH3 compared to the level of HCN, whereas higher-ranking (bituminous) coal has been shown to produce only HCN [471]. Studies by Winter et al. [715] have shown that for bituminous coal, using an HCN/NH3 partition ratio of 9:1 gave better NOx predictions when compared to measurements than specifying only a single intermediate species. Liu and Gibbs [386] work with woody-biomass (pine wood chips), on the other hand, has suggested an HCN/NH3 ratio of 1:9 due to the younger age of the fuel.
In total, the above work suggests the importance of being able to specify that portions of the fuel nitrogen will be converted to both HCN and NH3 intermediates at the same time. In Ansys Fluent, fuel nitrogen partitioning can be used whenever HCN or NH3 are intermediates for NOx production, though it is mainly applicable to solid fuels such as coal and biomass. The reaction pathways and source terms for HCN and NH3 are described in previous sections.